What is nanotechnology
Nanotechnology may sound futuristic, but it's already making a big impact on our daily lives, especially in the field of surface coatings. These coatings help protect things like walls and cars.
​
At the heart of nanotechnology are tiny particles called nanoparticles. They're incredibly small, measuring between 1 to 100 nanometers (a nanometer is one billionth of a meter). To put it in perspective, the width of a human hair is about 80,000 to 100,000 nanometers.
​
Scientists and engineers are using nanotechnology to manipulate and engineer these tiny materials to create amazing advancements that benefit us in many ways.

Microtechnology and nanotechnology are fields that work with very small sizes, ranging from millimeters to picometers. While both fields have their own characteristics, nanotechnology is particularly remarkable because it can manipulate atoms and molecules at the nanoscale.
​
Micrometres (µm): Microtechnology is excellent at the micrometre scale, allowing for the production of tiny components and devices. However, it becomes more challenging to control individual molecules and atoms at this level.
​
Nanometers (nm): Nanotechnology takes us to the nanometer scale, where we can manipulate individual molecules and atoms. This precise control enables the creation of nanomaterials with unique and customizable properties.
​
Picometers (pm): At the picometer scale, scientists can manipulate the positions of atomic nuclei. This unprecedented level of control opens up possibilities for designing quantum dots and other nanostructures used in quantum computing and advanced materials.
Nano in surface treatment (coating)
In the vast field of nanotechnology, material science is a prominent area of research and application. Scientists and engineers explore the properties of nanoscale materials to uncover their hidden potentials. One fascinating aspect of material science is the study of hydrophobicity, which plays a crucial role in developing advanced materials with unique characteristics and functionalities.
​
Hydrophobicity refers to a material's ability to repel water. Hydrophobic materials have the remarkable property to resist wetting and interaction with aqueous substances. This ability offers numerous practical applications in sectors ranging from textiles and coatings to consumer electronics and biomedical devices.
​
Surface modifications are prevalent in material science to achieve hydrophobicity. Scientists can treat or coat material surfaces with hydrophobic substances or nanostructures to alter their surface energy, making them less prone to water wetting. Deposition of thin layers of nanoparticle coatings, such as silicones or fluoroalkyl compounds, creates a hydrophobic barrier. Surface patterns at the nanoscale, achieved through nanolithography or self-assembly processes, further enhance hydrophobicity.
​
The rise of superhydrophobic materials, made possible by nanotechnology, has offered unparalleled water-repellent capabilities. These materials possess a unique hierarchical structure with nano-sized protruding features on a microscale rough surface. This combination creates air pockets that prevent water from directly contacting the surface, resulting in an excellent water-repelling effect.
​
Beyond hydrophobicity, nanotechnology has also facilitated advances in other material science properties, such as anticorrosion, anti-fouling, and self-healing abilities. The ability to engineer materials at the nanoscale allows scientists to tailor their properties and overcome traditional limitations.
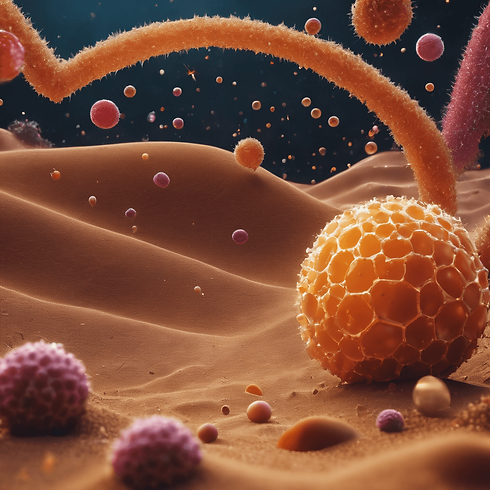
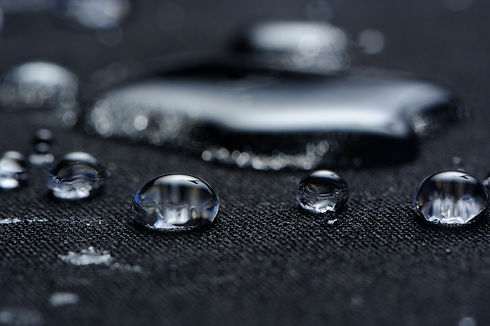
Hydrophobic coatings
Hydrophobic coatings are engineered to repel water by utilizing nano-material structures that create a surface coating. This unique structure minimizes contact between water droplets and the surface, allowing them to smoothly cascade away and carry dirt and contaminants with them, thereby maintaining surface cleanliness.
​
Hydrophobic Coatings
These coatings offer several benefits when rooted in nanotechnology. They provide water repellency, self-cleaning properties, and enhanced durability. As a result, they find applications across various industries, leading to the development of more efficient and environmentally-conscious products and processes.
​
The Lotus Effect
The Lotus Effect, a natural phenomenon observed in lotus leaves, serves as inspiration for superhydrophobic coatings. The micro and nanostructures found on lotus leaves create a self-cleaning effect by repelling water and maintaining cleanliness.
​
Contact Angle
In the realm of superhydrophobic coatings, the contact angle is a fundamental concept in surface science and fluid dynamics. It measures the angle formed between a liquid droplet and a solid surface at their point of contact. The contact angle plays a pivotal role in determining the water-repellent properties of superhydrophobic coatings.
Superhydrophobicity
Superhydrophobic coatings, inspired by the lotus effect, are engineered to exhibit extreme water repellency. These coatings create a nanostructured surface that allows water droplets to form high contact angles, typically greater than 150 degrees. This high contact angle results in a self-cleaning effect, as water beads up and easily rolls off the surface, carrying dirt and contaminants with it.
​
The unique properties of superhydrophobic coatings offer several benefits across various industries. Here are some key advantages:
​
Water repellency
Superhydrophobic coatings effectively repel water, preventing it from wetting the surface. This is particularly beneficial in applications where water contact can cause damage or affect performance, such as electronic devices or outdoor equipment.
​
Self-cleaning
The self-cleaning properties of superhydrophobic coatings mean that dirt, dust, and other contaminants are easily washed away by water droplets. This results in cleaner surfaces and reduces the need for frequent cleaning and maintenance.
​
Enhanced durability
The water-repellent nature of superhydrophobic coatings helps protect surfaces from water damage, corrosion, and staining. This leads to improved longevity and durability of coated materials.
​
Energy efficiency
In some cases, superhydrophobic coatings can aid in energy efficiency by reducing frictional drag caused by water. This can have implications in various industries, including transportation and renewable energy.
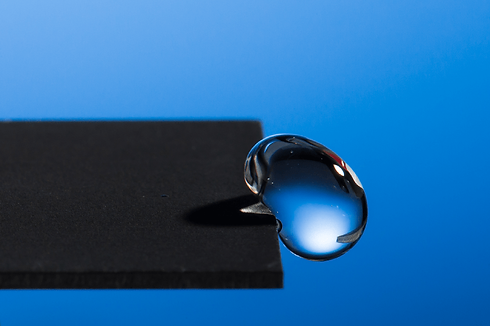

"The Lotus Effect"
The "Lotus Effect" is a fascinating natural occurrence that highlights the incredible ability of lotus leaves to repel water. Scientists and engineers have been inspired by this effect to create superhydrophobic materials that imitate the lotus plant's efficient water-repellent properties.
​
Lotus leaves have a unique self-cleaning mechanism. Their surfaces are covered in small bumps or spheres coated with wax, creating a structured and hydrophobic texture. This combination of surface roughness and the waxy coating makes it difficult for water droplets to stick to the surface. Instead, the droplets form round beads that effortlessly roll off the leaf.
​
This natural adaptation benefits the lotus plant in several ways. It keeps the leaves clean by preventing dirt and contaminants from sticking, and it allows sunlight to reach the chloroplasts unimpeded for efficient photosynthesis. The Lotus Effect showcases nature's ingenuity and serves as a model for developing practical materials with many applications across different industries.
Contact angle
The contact angle is typically measured as the angle formed between the tangent line at the point of contact on the solid surface and the two sides of the liquid droplet. It can be categorized into three primary regimes:
Acute angle (θ < 90 degrees): In this scenario, the liquid wets the surface, signifying that it spreads out and adheres to the surface. A contact angle measuring less than 90 degrees indicates hydrophilicity, where the liquid is attracted to the surface and tends to spread across it.
​
Right angle (θ = 90 degrees): This is referred to as the "Young-Laplace contact angle." When the contact angle precisely measures 90 degrees, it indicates that the liquid neither wets nor repels the surface but maintains a somewhat spherical shape.
​
Obtuse angle (θ > 90 degrees): An obtuse contact angle signifies that the liquid is repelled by the surface, forming a more spherical shape. A contact angle exceeding 90 degrees indicates hydrophobicity, where liquid beads adhere minimally to the surface.
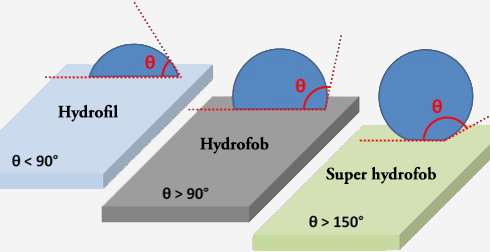
Source: Noxnatura
Scanning Electron Microscope (SEM)
A Scanning Electron Microscope (SEM) serves as a potent imaging instrument utilized for high-resolution examination and analysis of surface morphology and composition in various materials at the nanoscale. SEM operates by employing a focused electron beam to scrutinize the object's surface, furnishing comprehensive insights into its topographical features and elemental composition.
Applications in nanoscale observation
SEM plays a vital role in the characterization of various nanomaterials, such as nanoparticles, carbon nanotubes (CNTs), and nanowires, allowing scientists to examine their dimensions, configurations, and spatial dispersion.
​
Within the realm of nanotechnology, SEMs are indispensable tools for visualizing and assessing nanostructures, such as graphene sheets, quantum dots, and nanocomposites. They play a pivotal role in advancing research and ensuring the quality control of nanodevices and nanomaterials.
In the field of biology and life sciences, SEM serves as a valuable resource for exploring biological specimens at the nanoscale, encompassing cells, tissues, and microorganisms. It provides intricate insights into cellular structures and subcellular organelles.
Furthermore, SEM is instrumental in materials science, facilitating the examination of the surface topography of various materials, including metals, ceramics, and polymers, at the nanoscale. This enables researchers to analyze features like cracks, crystal grains, and defects with precision.